GE2R
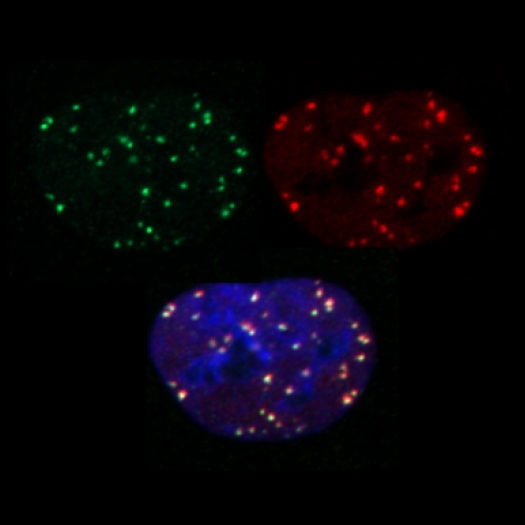
marquage yH2AX, 53BP1 et merge en tryptique
marquage yH2AX, 53BP1 et merge en tryptique
Genome Editing, DNA Repair and cellular Responses
RESEARCH OVERVIEW
Eukaryotic cells have developed diverse repair pathways to resolve DNA damage and the faithful repair is crucial to maintain genome integrity. On the other hand, genome editing with custom nucleases take advantage of these pathways to produce permanent genetic changes and is now a major strategy for investigating genome organization and gene function, creating animal models of disease and improving gene therapy. Our team is interested in studying DNA repair pathways, their roles in genome editing as well as in different biological situations, taking advantage of the opportunities raised by programmable nucleases.
Our projects are focused on two major objectives:
Cells have the capacity to deal with a wide range of DNA lesions, and genome editing, which always starts with introduction of a targeted lesion, further relies on cellular DNA repair to achieve genome modification. The ultimate goal of genome editing is to produce a desired sequence at high rates, with very few other mutations.
During the last years, CRISPR-Cas nucleases have been used to generate genome editing after introduction of a double-strand break (DSB) at the target site, which is repaired by either homology-directed repair (HDR), or end-joining repair. Precise genome editing i.e. programmed sequence modification can be performed following HDR, copying template DNA carrying the sequence change of interest (called donor DNA). However, end-joining repair pathways, canonical non-homologous end-joining (cNHEJ) and micro-homology-mediated end-joining (MMEJ), prevail over HDR, generally making HDR-based genome editing poorly efficient in many systems. The manipulation of DSB repair pathways is an important way to favor obtaining the desired sequence compared to other modifications.
Second generation of genome editing approaches have been described, namely base editing and prime editing, and allow DSB-free and donor-free genome editing. They are based on the use of fusion proteins between a Cas9 nickase and a DNA deaminase or a reverse transcriptase associated with an extended guide RNA (called pegRNA, for prime editing guide RNA), respectively. They can allow to obtain efficient and precise sequence changes in specific conditions: for base editors, precision mainly depends on the target sequence, while for prime editors (PE), efficiency strongly varies among cell types and types of edits.
A central goal of our team is to increase the efficiency of precise genome editing in different experimental contexts and to better understand and control the mechanisms of DNA repair involved. We have worked on direct targeting of HDR components at the DSB in order to favor HDR (6).
Our aims are now: (i) to characterize new players in the MMEJ pathway that appears to play a predominant role in repair of DSBs inflicted by CRISPR nucleases (11) and exploit them to improve genome editing; (ii) to overcome the current limitations of the PE approach.
These last few years, we developed some gene editing tools and successfully used them in various biological systems, including various animal models for functional studies (2) and human cells for gene therapy approaches (4). This expertise is transferred to the TACGENE facility and offered as a service to academic labs.
Figure 1: Genome editing strategies with CRISPR-Cas systems.
Genome editing approaches, DNA repair pathways involved and type of modifications achieved are indicated (MMR: MisMatch Repair, BER: Base Excision Repair, HDR: Homology-directed repair; MMEJ: Microhomology-mediated End Joining; NHEJ: Non-Homologous End-Joining).
We have chosen to expand our studies of DNA repair to tardigrades, a novel in vivo model organism well-known for its tolerance to extreme environments. Tardigrades are resistant to a great variety of harsh environments, including conditions that induce high levels of DNA damage. The study of tardigrades may therefore pave the way to identification of new proteins and pathways that handle DNA damages. Interestingly, a tardigrade protein called Dsup, which interacts with nucleosomes and can protect them from DNA damage, has been identified and is likely involved in this extreme resistance. However, the Dsup gene is only found in some tardigrades (within the Hypsibiidae superfamily), suggesting other genes and mechanisms could be involved.
Our goal is to examine the molecular basis of tardigrade genome tolerance as well as cell type-specific mechanisms, by identification of candidate genes and analysis of regulation of DNA repair pathways in different tardigrades species, using functional and comparative genomics approaches. We will also explore possible applications of tardigrade proteins in heterologous organisms.
By transcriptome analysis in response to ionizing radiation in three tardigrade species, we have recently identified a novel gene which we named tardigrade DNA damage response gene 1 (TDR1) that is only found in tardigrades and may play a key role in their DNA damage resistance. We have therefore started to characterize its mechanisms of action (1).
Finally multiple forms of reproduction have been reported in tardigrades, asexual reproduction by parthenogenesis or sexual reproduction, and we propose to explore this fascinating aspect of tardigrade biology and its consequences on tardigrade adpatation, by comparative genomics approaches, in association with cellular and molecular characterization of germ cell development and meiosis.
Figure 2: Nuclear localization of TDR1 in Hypsibius exemplaris tardigrades
mCherry and HeTDR1-mNeonGreen expression plasmids under control of the HeActin promoter were delivered into adult H. exemplaris tardigrades. Confocal imaging at day 8 post-delivery shows nuclear localization of HeTDR1-mNeonGreen fusion protein.
M. Anoud, E. Delagoutte, Q. Helleu, A. Brion, E. Duvernois-Berthet, M. As, X. Marques, K. Lamribet, C. Senamaud, L. Jourdren, A. Adrait, S. Heinrich, G. Toutirais, S. Hamlaoui, G. Gropplero, I. Giovannini, L. Ponger, M. Gèze, C. Blugeon, Y. Coute, R. Guidetti, L Rebecchi, C. Giovannangeli, A. De Cian, J-P. Concordet. Comparative transcriptomics reveal a novel tardigrade specific DNA binding protein induced in response to ionizing radiation, eLife (accepted), bioRxiv 2023.09.08.556854; doi: https://doi.org/10.1101/2023.09.08.556854
Rosello M, Serafini M, Mignani L, Finazzi D, Giovannangeli C, Mione MC, Concordet JP, Del Bene FC. Disease modeling by efficient genome editing using a near PAM-less base editor in vivo. Nat Commun. 2022 Jun 15;13(1):3435. doi: 10.1038/s41467-022-31172-z. PMID: 35701478
Geny S, Pichard S, Poterszman A, Concordet JP. Gene Tagging with the CRISPR-Cas9 System to Facilitate Macromolecular Complex Purification. Methods Mol Biol. 2021; 2305:153-174. doi: 10.1007/978-1-0716-1406-8_8. PMID: 33950389.
Weber L, Frati G, Felix T, Hardouin G, Casini A, Wollenschlaeger C, Meneghini V, Masson C, De Cian A, Chalumeau A, Mavilio F, Amendola M, Andre-Schmutz I, Cereseto A, El Nemer W, Concordet JP, Giovannangeli C, Cavazzana M, Miccio A. Editing a γ-globin repressor binding site restores fetal hemoglobin synthesis and corrects the sickle cell disease phenotype. Sci Adv. 2020 Feb 12;6(7):eaay9392. doi: 10.1126/sciadv.aay9392. PMID: 32917636; PMCID: PMC7015694.
Concordet JP, Haeussler M. CRISPOR: intuitive guide selection for CRISPR/Cas9 genome editing experiments and screens. Nucleic Acids Res. 2018 Jul 2;46(W1):W242-W245. doi: 10.1093/nar/gky354. PMID: 29762716; PMCID: PMC6030908.
Charpentier M, Khedher AHY, Menoret S, Brion A, Lamribet K, Dardillac E, Boix C, Perrouault L, Tesson L, Geny S, De Cian A, Itier JM, Anegon I, Lopez B, Giovannangeli C, Concordet JP. CtIP fusion to Cas9 enhances transgene integration by homology-dependent repair. Nat Commun. 2018 Mar 19;9(1):1133. doi: 10.1038/s41467-018-03475-7. PMID: 29556040; PMCID: PMC5859065.
Hosseini ES, Nikkhah M, Hamidieh AA, Fearnhead HO, Concordet JPC, Hosseinkhani S. The Lumiptosome, an engineered luminescent form of the apoptosome can report cell death by using the same Apaf-1 dependent pathway. J Cell Sci. 2020 May 27;133(10):jcs242636. doi: 10.1242/jcs.242636. PMID: 32461338.
de Oliveira VC, Gomes Mariano Junior C, Belizário JE, Krieger JE, Fernandes Bressan F, Roballo KCS, Fantinato-Neto P, Meirelles FV, Chiaratti MR, Concordet JP, Ambrósio CE. Characterization of post-edited cells modified in the TFAM gene by CRISPR/Cas9 technology in the bovine model. PLoS One. 2020 Jul 10;15(7):e0235856. doi: 10.1371/journal.pone.0235856. PMID: 32649732; PMCID: PMC7351154.
Babin L, Piganeau M, Renouf B, Lamribet K, Thirant C, Deriano L, Mercher T, Giovannangeli C, Brunet EC. Chromosomal Translocation Formation Is Sufficient to Produce Fusion Circular RNAs Specific to Patient Tumor Cells. iScience. 2018 Jul 27;5:19-29. doi: 10.1016/j.isci.2018.06.007. Epub 2018 Jun 19. PMID: 30240643; PMCID: PMC6123901.
Concordet JP, Haeussler M. CRISPOR: intuitive guide selection for CRISPR/Cas9 genome editing experiments and screens. Nucleic Acids Res. 2018 Jul 2;46(W1):W242-W245. doi: 10.1093/nar/gky354. PMID: 29762716; PMCID: PMC6030908.
Momose T, De Cian A, Shiba K, Inaba K, Giovannangeli C, Concordet JP. High doses of CRISPR/Cas9 ribonucleoprotein efficiently induce gene knockout with low mosaicism in the hydrozoan Clytia hemisphaerica through microhomology-mediated deletion. Sci Rep. 2018 Aug 6;8(1):11734. doi: 10.1038/s41598-018-30188-0. PMID: 30082705; PMCID: PMC6078951.
Renaud J-B., Boix C., Charpentier M., De Cian A., Cochennec J., Duvernois-Berthet E., Perrouault L., Tesson L., Edouard J., Thinard R., Cherifi Y., Menoret S., Fontanière S., de Crozé N., Fraichard A., Sohm F., Anegon I., Concordet J-P. & Giovannangeli C. (2016) Improved Genome Editing Efficiency and Flexibility Using Modified Oligonucleotides with TALEN and CRISPR-Cas9 Nucleases. Cell Reports. 14,9:2263-72.